First Flight Attempt and Redemption
This week had many major developments for the semester project. Finally, the weather is good enough to actually begin field work. To begin the week, on Monday, we had the opportunity to get a small team together to fly the M600 UAS. Being that it would be it's maiden flight, a small area was chosen. The goal was to fly around the amphitheater in our target park to create a 3D model.We set out Aeropoint ground control markets around the amphitheater near permanent locations to assist in the orthorectification (Figure 1). Points were also placed along topographic gradients to help improve the precision of information in the Z-axis.
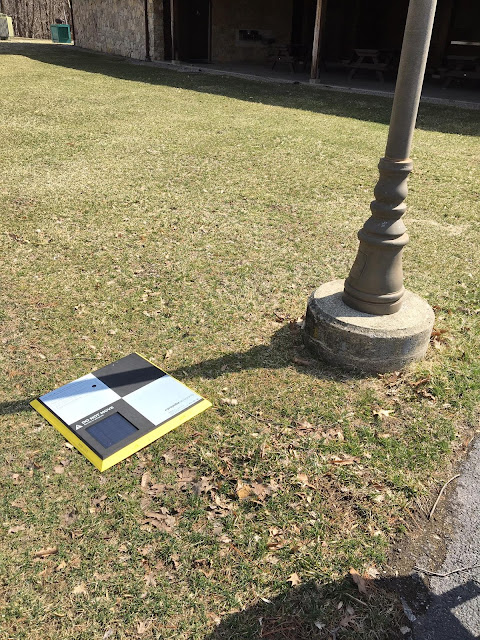 |
Figure 1: Ground control point placed near light post. |
After setting out ground control points, we began setting up the aircraft (Figures 2 & 3). After setting up the aircraft, we unfortunately ran into an issue with calibration of our compass. It was unresponsive to the calibration exercises. Compass health, as well as the prompts that were being displayed, were indicative of significant interference. In our opinion, the most likely culprit were underground power lines. It is likely that power lines were present as nearby structures did not have obvious above ground power connections, but certainly had access to electricity. After moving the aircraft to the grass (Figure 3), the calibration was successful with nominal compass health. However, the RGB sensor was unresponsive to the aircraft electrical bus system. We knew that the issue was somewhere along the sensor mount or connection because we briefly rewired the sensor to the landing gear bus cable, with still no response. While this was a positive note because it was indicative of power issues isolated to the sensor, it still indicated a malfunction.
 |
Figure 2: M600 aircraft being set up for flight. |
 |
Figure 3: Front view of the aircraft. The aircraft moved to grass to avoid interference. |
Unfortunately, we were not able to get the sensor to function properly in the field. We did not have requisite tools to open the aircraft airframe to check on the electrical distribution system. This indicates that we need to construct an appropriate field-kit for this aircraft. However, the fact that we did not open the aircraft's internals worked to our advantage. Later Monday afternoon, without any changes to the aircraft at all, the sensor activated properly. We are not sure what changed in the electrical distribution for the sensor to start. Our best estimates is that weather played a factor. It was very cold the morning we attempted to fly the m600, but not out of the aircraft or sensor's appropriate range. While the temperature range should have allowed for proper sensor function, we believe that condensation may have occurred, disabling the sensor's connectivity to the aircraft.
Regardless of the actual cause of disconnect, we managed to test fly the aircraft on a Purdue FNR forest test plot in the Martell Forest Thursday afternoon. The aircraft performed as planned in two different flights over a black walnut stand. The goal was to create a 3D mesh, very similar to the original goal with the amphitheater. Data is yet to be processed for the flight, but at first glance it appears to be of quality. Data examples will be shared in the following week's blog post.
C-Astral Maiden Flight
The following morning presented the first opportunity to fly the C-Astral Bramor PPX aircraft. We met early in the morning in our lab space to plan, organize, pack equipment, and head to the amphitheater park. The goal was to map the entirely of the internals to the park at the highest resolution possible without risking flight safety in any capacity. For this reason, our 42MP RGB sensor was chosen. Based on training extent, the following roles were assigned to crew members:
1) Evan (Myself) - Pilot-in-Command
2) Kyle (Sheehan) - Co-Pilot
3) Ian Willey - Equipment Manager
4) Todd Horn - Primary Visual Observer
5) Thomas Gonya - Secondary Equipment Manager
6) Ryan Ferguson - Photographer & Observer Control
7) Dylan McQueen - Ground Control Lead
8) Krysta Rolle - Ground Control Support and Assistant Visual Observer
Normally we would likely not have everyone present, but being the maiden flight, everyone played a role. During the checklist, everyone stepped in to provide guidance where needed as the four required crew members performed their roles (Figure 4). This helped ensure nothing was missed.
 |
Figure 4: The majority of the crew assisting in checklist steps. |
Being the pilot, my primary task was to complete all steps regarding the digital elements of the checklist. This includes manipulating the flight control devices such as the control tablet and communication transmitter. This also includes setting model, checking critical waypoint settings, and checking aircraft sensor conditions (Figure 5).
 |
Figure 5: Myself, manipulating the flight controls before the motor test step of the checklist. |
By the time of aircraft launch, we had at least triple checked each flight setting. These included the extremely important takeoff, home, rally, parachute, and landing points. Each of these points are responsible for how the aircraft steps-up and steps-down from altitude before and after the survey mission. These steps are extremely critical to a safe and successful mission, and the PIC's primary task is to get the aircraft between these points as best as possible. To do this, close control of altitude, speed, turn radius, and general awareness of environmental factors is needed.
The aircraft launch went smoothly after a slightly concerning dip after takeoff. The likely reason is because we chose launch direction mostly based on obstacles, not strictly wind direction (Figures 6 & 7).
 |
Figure 6: Aircraft immediately after takeoff. |
 |
Figure 7: Aircraft in initial climb. |
Once in flight, the aircraft circled the takeoff point in a climb to 75 meters. This height was low enough to deploy the parachute if needed, and high enough to safely avoid all obstacles with room to spare. While the aircraft was in this stage of flight, the observer crews dispersed to a variety of locations throughout the park. These areas provided great visual line of sight with the aircraft, as well as the surrounding environment, from all areas. My Co-Pilot, Kyle, acted as my voice in our group's radio communication network.
After time was provided for crews to spread out and for the flight crew to observe the flight condition and status of the aircraft, the mission was commenced. The aircraft was tasked with climbing to 120 meters for the survey at a cruise speed of 16 m/s. Strangely, the aircraft actually overshot the cruising altitude to what was set in altitude override, despite that setting being not engaged. This was immediately corrected by engaging the altitude override setting, and setting the aircraft to cruise at the requisite 120 meters. In flight, the aircraft flew the survey flawlessly (Figure 8). We also realized that the aircraft was visible at the set altitude from the launch site almost the entire time. A single remote observer crew, or perhaps two if available, would be more than sufficient.
 |
Figure 8: Aircraft in survey mission, passing over photographer, Ryan Ferguson. |
At the end of the survey, about 25 minutes later, the aircraft returned to the rally point. The rally point was to the South of the landing and launch points. Once we decided it was time to the land, the aircraft entered landing mode. From there, the aircraft began a spiraling decent to 55 meters. Strangely, the aircraft appeared to be much lower than the set height, and it looked like it was narrowly missing trees that were no more than 20 meters tall at most. This was almost certainly because of perception, as we are all used to much smaller fixed wing aircraft, and the aircraft was likely quite high over the trees.
Parachute deployment was perfect, but the aircraft landed quite far away from the supposed landing site. Our assumption is that the slight tailwind was greater than expected slightly at altitude, and thus caused the aircraft to have to slow down further for successful deployment and have a longer parachute glide distance.
Overall the flight lasted about 34 minutes with 460 images taken (figure 9). The aircraft traveled almost 35 km, and we later confirmed that the surveyed areas was about 75 hectares. Immediately returning to lab, the aircraft's data was inserted into the pre-processing pipeline with PPK correction. Some confusion has arisen with the PPK corrected data, as the initial orthomosaics appear to have spatial mismatch issues with basemap images beyond what is acceptable. Further explanation will be covered in next week's post which will be very data centered. Examples of the data products from this flight, as well as flights next week, including an explanation of our pre-processing pipeline, will be covered.
 |
Figure 9: Flight summary produced post flight. |
Changes for Next C-Astral Flight
Some major considerations will be made by next week. The first, we will no longer prioritize obstacle issues over wind direction for launch. The aircraft has a relatively low stall speed, so it is understandable how a slight tailwind would be conducive to a dramatically increased takeoff distance before climb out. This needs to be factored in further in the future. We will try to better understand the purpose of altitude override and why, even when disabled, it influenced flight altitude. We will also add a checklist item to ensure that this step is set to desired cruise altitude. This should remove any issues the function may cause. After the survey is complete, the landing point, and likely the rally point, will be reevaluated based on winds better. While the aircraft is capable of landing with the wind, and may be necessary some times, a headwind should provide for more controlled performance. When flying at the same field, we will rally out in front towards where the takeoff point was, so that headwind landings are more likely throughout the spring. Despite these lessons, this flight was a successful maiden and data collection flight with our flagship platform. Many more data collection missions will be happening throughout the following month with this platform. See figure 10 to see an examples of notes taken in the field that correlate to this section of the blog post.
 |
Figure 10: Field notes for flight and this final section. |